3.1 Soil Water Content
When the United States National Aeronautics and Space Administration (NASA) was building the Phoenix Mars Lander to explore the surface of Mars, the project leaders contacted soil physicists to create a special sensor for the rover’s robotic arm (Fig. 3‑1). The purpose of that sensor was to test for the presence of water in the Martian soil. The fascinating story of the resulting partnership between NASA and soil scientists is recounted in this video. The NASA scientists knew that finding water on Mars was key to answering questions about the possibility of life on that hostile planet. Water is the life-blood of the soil. Almost all terrestrial life and almost all soil biological, chemical, and physical processes are influenced by the water content of the soil.
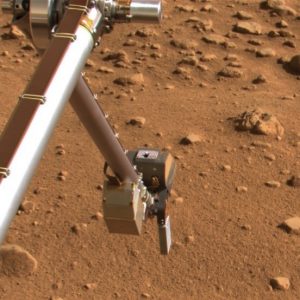
3.1.1 Soil Water Content Terminology
As you continue to study soil physical properties and processes, you will need to know and use the following key terms related to soil water content.
- Gravimetric water content (θg) is the mass of water per unit mass of oven-dry soil particles. It has dimensions of mass over mass and may be written as a unitless decimal, as a percentage, or as a decimal with units of kg kg-1 or g g-1. We will use the latter in this book. For mineral soils it ranges from approximately 0 to 0.60 g g-1. Organic soils may have higher values.
- Volumetric water content (θ) is the volume of water per unit volume of soil. It has dimensions of volume over volume and may be written as a unitless decimal, as a percentage, or as a decimal with units of m3 m-3 or cm3 cm-3. We will use the latter in this book. For mineral soils it ranges from approximately 0 to 0.60 cm3 cm-3. Organic soils may have higher values. When gravimetric water content is known, the volumetric water content can be calculated using the soil bulk density (ρb) and the density of water (ρw). This relationship is further explained in this video.
(Eq. 3-1)
Soil water storage (S) is the equivalent depth of soil water contained in a soil layer of a specified thickness (Δz). It has dimensions of length and may be written as cm or mm. If the volumetric water content of the layer is known, then the soil water storage is calculated by S = θ × Δz.
3.1.2 Soil Water Content Measurement Techniques
Knowing the amount of water contained in the soil is important, not only in the search for life on Mars, but also for understanding and managing life on Earth. Researchers in disciplines such as soil science, hydrology, ecology, agronomy, meteorology, and civil engineering often need accurate measurements of soil water content; as do farmers, horticulturalists, turf managers, and government agencies responsible for managing natural resources. Since around the end of the World War II, researchers have been continually developing new methods for measuring soil water content to meet the needs of these diverse user groups [1]. We will briefly consider a few of those measurement techniques here.
The simplest and oldest method for measuring soil water content is called the gravimetric method. In this method, a soil sample is weighed, then oven-dried at 105°C to a constant weight and the final dry weight is recorded. This drying temperature is above the boiling temperature for water but below temperatures at which soil organic matter would be lost by heating. If the volume of the soil sample is unknown, then only the gravimetric water content can be determined using this method. If the volume of the sample is known, then the bulk density and volumetric water content can also be determined. In practice, a fixed drying time of 24 hours is often used because it is more convenient than repeatedly weighing the sample to determine if a constant weight has been reached. The primary requirements for this method then are an accurate balance and an oven that can safely hold a constant temperature unattended for 24 hours.
In contrast to the simplicity of the gravimetric method, powerful new methods of measuring and monitoring soil water content involve a diverse array of cutting-edge technologies. Now satellites orbit the Earth carefully mapping the emission of electromagnetic radiation from the land surface in the microwave wavelength range, and these data are used to estimate the volumetric water content of the 0-5 cm soil layer around the globe with ~9 km spatial resolution [2, 3]. Researchers are also learning to extract information about soil water content from the satellite-generated signals received by antennas of Global Positioning Systems because a portion of those signals comes from reflections off the land surface [4, 5].
Meanwhile, neutrons generated by cosmic-rays are detected with stationary or mobile neutron counters at the land surface and used to estimate volumetric water content of the surface soil to a depth of up to 40 cm with a spatial resolution of ~400 m [6, 7]. For higher spatial resolution, researchers deploy fiber optic distributed temperature sensing systems with or without active heating capabilities. These systems can measure soil temperatures at 1-m resolution and 1-min intervals along paths of 1-km length or greater, and the soil temperature data can be used to infer soil volumetric water content [8, 9]. Fig. 3‑2 shows some of the equipment in a field experiment called the Marena, Oklahoma, In Situ Sensor Testbed (MOISST) where many of the established and emerging soil moisture measurement technologies are inter-compared [10].
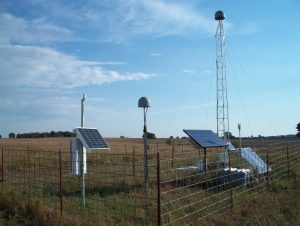
Add to these techniques the steady progression of new electromagnetic and thermal sensors that permit long-term, automated monitoring of soil volumetric water content at a single point [11] , a progression that began with the seminal work of Topp et al. [12] who used a technique called time domain reflectometry (TDR). You can see that development of improved methods for measuring soil water content has been a major focus in soil physics and related disciplines, and you can understand why soil water content data are becoming increasingly available around the world [13]. One of the great opportunities for researchers today is to find creative ways to use these data for societal benefit whether for improved drought monitoring, more accurate streamflow forecasting, increased wildfire preparedness, or a host of other potential applications [14-16].